4. Low speed high AOA characteristics
4.1 Lift-body LERX Canard configuration
Advanced modern fighters utilized research on detached vortices from the 1960s and 70s to gain excellent lift characteristics with their max lift coefficient peaking at around 1.6. They either employ conventional LERX configuration or canard configuration to accomplish this. The future fighter has higher requirements for max lift coefficient and the situation is further complicated by the fact that the use of twin vertical stabilizers is detrimental to lift (see figure 4.2). As a result the design team must raise the max lift coefficient to a whole new level. It will be difficult to realize this goal simply employing conventional LERX configuration or canard configuration.
It is beneficial to choose canard configuration from a high AOA pitch down control stand point(see figure 4.3). Blending lift body LERX characteristics with the conventional canard configuration to form a "lift body LERX canard configuration" will greatly enhance the max lift characteristics. Exploration of the lift body LERX canard configuration will solve three important technical issues. The first problem is the aerodynamic coupling between canards and medium sweep, medium aspect ratio wings. The second problem is the coupling between the canards, the LERX, and detached vortices generated by the wings. The third problem concerns the gains and losses of employing body lift on a canard configuration aircraft.
Traditionally close coupled canard configuration aircraft utilize constructive coupling between the canards and detached wing vortices to enhance the max lift coefficient. Only wings with large back-sweep angle and small aspect ratio could generate detached vortices that are powerful enough for the task. As a result most modern canard configuration fighter aircraft have a leading edge backsweep angle of around 55 degrees and an aspect ratio of around 2.5. For these aircraft, the canards could generate around a 3 to 4 times increase in max lift coefficient with respect to their wing areas. Ideally we hope to employ wings with medium leading edge backsweep angle and medium aspect ratio in order to improve lift characteristics over the entire AOA range. This wing shape, however, could not effectively generate leading edge detached vortices. Could the canards still attain their original lift enhancing effects? The answer is yes according to wind-tunnel tests. As the slope of the aircraft's lift curve increases, the lift enhancing capabilities of the canards are the same as those on traditional close coupled canard configuration aircraft (see figure 2). The key influence on aerodynamic coupling between the canards and medium back-sweep, medium aspect ratio wings should not be interference among detached vortices. Preliminary studies indicate that down-wash on the wings generated by the canards play a far greater role.
It is a well known fact that LERX could improve the max lift characteristics on medium back sweep, medium aspect ratio wings. In order to obtain even better lift characteristics, we should consider using both canards and LERX to create a canard-LERX configuration. Study shows that employing both canards and LERX not only retain the lift enhancing effects of the two mechanisms when they are used separately but also help achieve higher lift-coefficient (see figure 3). This means that there is beneficial coupling among the canards, LERX, and the wings.
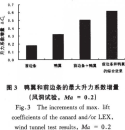
Blended wing lift body configurations could utilize lift generated by the aircraft's body to increase internal load and enhance stealth characteristics at relatively low costs to drag. Lift-body configurations have been adapted by many conventional configuration aircraft and achieved excellent results. Yet until now now canard configuration fighter utilized lift-body configuration. This isn't because aerodynamic experts failed to realize the tremendous advantage of the lift body configuration but the result of a canard configuration aircraft's need to place the canards above the aircraft's wings. It is difficult for lift-body configuration aircraft to satisfy this demand. Our experimental results indicate that although the canards on a canard-LERX configuration aircraft employing lift-body suffered a decrease in lift-enhancing effects, the overal lift characteristic of the aircraft was still superior to that of a canard-LERX aircraft not employing lift-body (see figure 4). Figure 5 shows the vortex generation on the wings and body of a lift-body canard configuration aircraft observed using laser scanning. It demonstrates that planes employing this configuration derive excellent lift characteristics not only from coupling among the canards, LERX, and detached vortices but beneficial interaction between the left and right detached vortices. The latter contribute to significant lift on the body of the plane and greatly contributed to the enhancement of lift characteristics. Figure 5 also indicates that the detached vortices primarily contribute to lift on the body and inner portions of the wings. Consequently, most of the lift produced under high AOA conditions are generated in the corresponding areas.
4.2 Canted vertical stabilizers
Vertical stabilizer design is an important consideration when it comes to future fighter configuration design. From a lateral stealth stand point, the vertical stabilizers should cant inward or outward to reflect incoming radar waves in other directions. The future fighter must be long and thin to accommodate for supercruise and as a result, the space between the vertical stabilizers couldn't be too wide. The twin stabilizers should cant outward in order to decrease destructive interference between the vertical stabilizers. Since the future fighter will fully utilize detached vortices to improve max lift coefficient, forward vortices will generate relatively high outward facing velocity airflow on the vertical stabilizers. Figure 6 shows the calculation results of a type of lift body LERX canard configuration fighter using n-s time average function. It indicates the limiting flow rate on the aircraft's rear once the vertical stabilizers are removed. The results indicate that the regional side slip angle at the location where vertical stabilizers are usually installed reaches around 15 degrees when the AOA is 24 degrees and the side slip angle is 0 degrees. If the back-sweep angles of the vertical stabilizers are sufficiently large, the enormous regional side slip angles could generate leading edge shed vortices on the external faces of the stabilizers and form low pressure regions. Regional sideslip angles will also increase the static pressure on the inner portions of the vertical stabilizers. As a result, the vertical stabilizers will become highly efficient lateral force surfaces which direct the lateral forces outwards. The lateral forces are projected in the direction of lift, with respect to the outward canting vertical stabilizers, and generate negative lift. Negative lift acting on the vertical stabilizers and rear body will both contribute to the undesirable pitch up torque. The high pressure region between the vertical stabilizers will form adverse pressure gradients on the body of the plane and negatively impact the stability of leading edge detached vortices. Since the vertical stabilizers are already highly loaded at 0 degree side slip angle, the yaw/roll stabilization efficiency of the vertical stabilizers will be decreased.
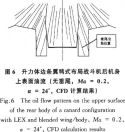
The negative impacts of vertical stabilizers as described above are closely associated with lift-enhancing measures and are, as a result, difficult to root out. Yet adjustment of the vertical stabilizer’s area, position, cant angle, and placement angle and improvement measures such as making slots on the rear body can minimize the negative impact of the vertical stabilizers. Ordinarily, the max lift reduction coefficient generated by the vertical stabilizers could reach around 0.4. We’ve managed to successfully lower it below 0.1 through experimentation.
Decreasing the vertical stabilizers’ area or even employing tailless configuration are directions worth studying. Their significance not only include improving low speed high AOA performance but also help improve stealth characteristics, lower drag within the entire flight envelope, decrease weight, and reduce cost. Implementing the tailless configuration requires the tackling of three major technical difficulties: replacing the stabilizers with another yaw control mechanism, installing sensitive and reliable side slip sensors, and implementing new flight control technology. As of now, these difficulties are being tackled one at a time. Relatively speaking, decreasing vertical stabilizers’ area and relaxing static yaw stability are more realistic options. Generally speaking, the relative size of the vertical stabilizers is around 20% to 25%. In or studies, utilizing all moving vertical stabilizers with 10% to 13% could still maintain basic yaw stability while retaining the vertical stabilizers’ function as yaw control mechanisms.